Morphology and Molecular Identification of Echinostoma revolutum and Echinostoma macrorchis in Freshwater Snails and Experimental Hamsters in Upper Northern Thailand
Article information
Abstract
Echinostome metacercariae were investigated in freshwater snails from 26 districts in 7 provinces of upper northern Thailand. The species identification was carried out based on the morphologies of the metacercariae and adult flukes harvested from experimental hamsters, and on nucleotide sequences of internal transcribed spacer 2 (ITS2) and nicotinamide adenine dinucleotide dehydrogenase subunit 1 (nad1) genes. Twenty-four out of 26 districts were found to be infected with echinostome metacercariae in freshwater snails with the prevalence of 40.4%. The metacercariae were found in all 6 species of snails, including Filopaludina martensi martensi (21.9%), Filopaludina doliaris (50.8%), F. sumatrensis polygramma (61.3%), Bithynia siamensis siamensis (14.5%), Bithynia pulchella (38.0%), and Anenthome helena (4.9%). The echinostome metacercariae found in these snails were identified as Echinostoma revolutum (37-collar-spined) and Echinostoma macrorchis (45-collar-spined) morphologically and molecularly. The 2-week-old adult flukes of E. revolutum revealed unique features of the cirrus sac extending to middle of the ventral sucker and smooth testes. E. macrorchis adults revealed the cirrus sac close to the right lateral margin of the ventral sucker and 2 large and elliptical testes with slight indentations and pointed posterior end of the posterior testis. The ITS2 and nad1 sequences confirmed the species identification of E. revolutum, and the sequences of E. macrorchis have been deposited for the first time in GenBank. The presence of the life cycle of E. macrorchis is a new record in Thailand and the snail F. doliaris as their second intermediate host seems to be new among the literature.
INTRODUCTION
Echinostomes have been found in the intestines of poultry and mammals (including humans) and to cause echinostomiasis [1,2]. The clinical symptoms in heavy infections are abdominal pain, diarrhea, weakness, and weight loss [3,4]. Destruction and erosion of villi also commonly occur in echinostome infections [5]. At least 23 species belonging to 10 genera are known to infect humans, i.e, Echinostoma, Echinochasmus, Acanthoparyphium, Artyfechinostomum, Echinoparyphium, Euparyphium, Episthmium, Himasthla, Hypoderaeum and Isthmiophora [6,7]. In Thailand, 4 echinostomes have been reported from humans, including Artyfechinostomum malayanum, Echinostoma revolutum, Echinostoma ilocanum, and Hypoderaeum conoideum [8], and 3 species have been recorded in poultry: Echinoparyphium recurvatum, E. revolutum, and H. conoideum [9,10]. Humans can be infected with echinostome metacercariae by eating raw snails, fish, or frogs [11,12]. In particular, freshwater snails can be the first and second intermediate host of echinostomes [13–16]. Many species of freshwater snails have been found to be infected with the metacercariae of echinostomes in Thailand, including Filopaludina spp., Anentome helena, Bithynia spp., and Eyriesia eyriesi [17–21]. The echinostome infections of snails have been recorded in some provinces, including Chiang Mai, Chiang Rai (Mae Lao district), and Lamphun [17–19,22]. However, there have been no studies of echinostome infections in snails from other provinces in Upper Northern Thailand. In addition, the recovered echinostome metacercariae in previous studies from some areas in Northern Thailand have not been identified to the species level [19,22].
Adult stages derived from the host experimentally infected with metacercariae are used for species identification. However, there are various factors, such as infective doses, immunological status, and age of the experimental host that can influence the establishment of adult echinostomes [23]. Meanwhile, the metacercarial stage is also difficult to identify because it is at larval stage not fully developed or matured. Recently, molecular genetics have been increasingly used to discriminate among morphologically similar parasites. PCR techniques have been applied to specific diagnosis of medically important echinostomes [24,25]. Moreover, DNA sequencing techniques potentially permit species, strains, and populations to be identified at the developmental stage of life. Many DNA regions have been used to distinguish among 37-collar-spined species of the genus Echinostoma and other echinostome species in the family Echinostomatidae [26–28]. In particular, the second internal transcribed spacer (ITS2) region and nicotinamide adenine dinucleotide dehydrogenase subunit 1 (nad1) gene have been used to identify both larval and adult stages of echinostomes [28–31].
In Northern Thailand, the identification of echinostome metacercariae in snails has been based either on obtaining the adult stage from experimental hosts or on molecular approaches on the metacercariae or adults [17,18]. Thus, in the present study, for confirmation of echinostome metacercariae originating from freshwater snails in Upper Northern Thailand, we aimed to study both the morphology of adult flukes and molecular characteristics of the metacercariae and adults. The prevalence of echinostome metacercariae in snails was determined based on the morphology of the metacercariae and also on the adult stage obtained from experimental hamsters. Nucleotide sequences of ITS2 regions and nad1 gene were also investigated. This study will be useful to demonstrate the epidemiological situation of echinostome infections in Upper Northern Thailand and to validate data for control and prevention programs.
MATERIALS AND METHODS
Ethical statement
All experimental hosts were managed according to the guidelines approved by the Institute of Animals for Scientific Purposes Development (IAD), and the relevant document (no. U1-07721-2561) was approved by the committee. The guidelines for animal care and use of animals for research, testing, biological production and teaching in the kingdom of Thailand were used according to the Animals for Scientific Purposes Act 2015.
Sample collection and investigation of metacercarial infection
Random samples of snails were collected between October 2017 and March 2019. The specimens were collected from 26 districts in 7 provinces of upper northern Thailand, including Chiang Mai, Chiang Rai, Lampang, Lamphun, Nan, Phare, and Phayao (Fig. 1). The metacercariae of echinostomes were investigated by the crushing method and then examined under a light microscope. The metacercariae were found on the pericardial cavity of the snail.
Morphological observations
The echinostome metacercariae were primarily identified based on the number of collar spines. The recovered metacercariae in each species of snails were experimentally fed to hamsters (Mesocricetus auratus) to obtain the adult stages. Two weeks following the onset of infection, the adult flukes were collected from the intestines of experimental hamsters. For morphological studies, the specimens were fixed in 4% formalin to be mounted on slides, stained with hematoxylin, dehydrated in ascending grades of alcohol, cleared using xylene, and mounted in Permount.
Amplification of ITS2 and nad1 regions
Genomic DNA of larval and adult stages were extracted by using a Chelex solution. In brief, 150 μl of 5% Chelex (Fluka) solution containing 10 μl of proteinase K (Sigma-Aldrich, St. Louis, Missouri, USA) at a concentration of 20 mg/ml was added to approximately 20 mg of trematode tissue. The sample was then heated at 55°C for 1 hr, followed by gentle vortexing and heating at 95°C for 30 min, again followed by gentle vortexing. The mixture was centrifuged at 13,000 g for 1 min. The supernatant was collected and stored at −20°C until it was to be used.
The ITS2 region was amplified using a forward 3S primer (5′-GGT ACC GGT GGA TCA CTC GGC TCG TG-3′) and reverse BD2 primer (5′-TAT GCT TAA ATT CAG CGG GT-3′). The PCR conditions were as follows: 2 min initial denaturation at 94°C, followed by 35 cycles of 1 min DNA denaturation at 94°C, 1 min primer annealing at 57°C, 1 min extension at 72°C, and a final extension at 72°C for 7 min.
The nad1 gene was amplified by using the primers, JB11 (5′-AGA TTC GTA AGG GGC CTA ATA -3′) as the forward primer and JB12 (5′-ACC ACT AAC TAA TTC ACT TTC -3′) as the reverse primer. The PCR conditions were as follows: 2 min initial denaturation at 94°C, followed by 39 cycles of 30 sec DNA denaturation at 94°C, 20 sec primer annealing at 48°C, 1 min extension at 72°C, and a final extension at 72°C for 10 min.
Agarose gel electrophoresis with DNA-Dye NonTox staining was used to visualize ITS2 and nad1 products under ultraviolet light. Both ITS2 and nad1 PCR products were purified and subjected to sequencing. The query sequences of ITS2 (MT314338–MT314343) and nad1 (MT815858–MT815862 and MT982427) were aligned using ClustalW with GenBank sequences of relative species in family Echinostomatidae and out group (Fasciola gigantica: Fasciolidae). The phylogenetic trees of ITS2 and nad1 were constructed using MEGA version 6.0 based on neighbor-joining (NJ) and maximum-likelihood (ML) methods. The statistics supported for the branches were tested using 1,000 bootstrap replicates.
RESULTS
Prevalence and intensity of echinostome metacercariae in snails
Our results showed that 1,496 (40.4%) of 3,705 freshwater snails were infected with echinostome metacercariae. The echinostome metacercariae were detected in 24 districts with the exception of Pua in Nan and Mae Chai in Phayao Provinces (Table 1). The highest infection was found in Rong Kwang district from Phare Province with the prevalence of 100%. A total of 3,705 snails were collected from this study, and they were classified into 3 families and 6 species. The metacercariae were recovered from all 6 species of snails, including Filopaludina martensi martensi, Filopaludina doliaris, Filopaludina sumatrensis polygramma, Bithynia siamensis siamensis, Bithynia pulchella, and Anenthome helena. The prevalence and intensity of metacercarial infections in each snail were detailed in Table 2. The snails belonging to the family Viviparidae were infected more with echinostome metacercariae than 2 remaining families (Bithyniidae and Nassariidae). The highest prevalence was found in F. sumatrensis polygramma (64.0%), while the lowest prevalence was in A. helena (4.9%).
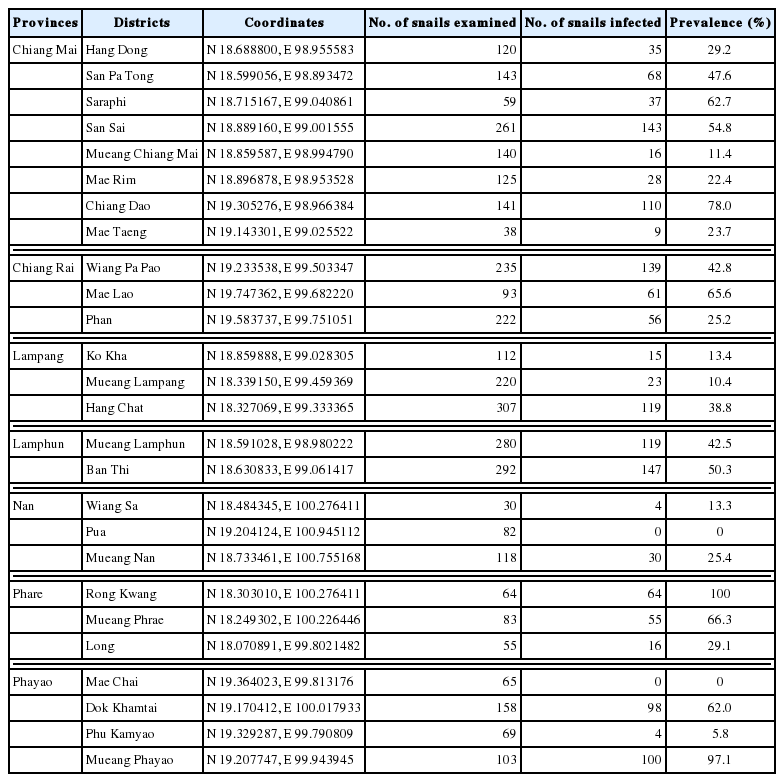
Locality of freshwater snails collected and the prevalence of echinostome infections in 26 sampling sites visited during 2017–2019 in upper northern Thailand
Morphological observations
The echinostome metacercariae were recovered in the pericardial cavity of the freshwater snails. They were spherical in shape. The cyst wall presented with an outer layer and an inner layer. The internal cyst contained the larva with collar spines and excretory granules. The echinostome metacercariae isolated from F. martensi martensi, F. sumatrensis polygramma, A. helena, B. siamensis siamensis, and B. pulchella revealed 37 collar spines, while those from F. doliaris showed 45 spines.
The metacercariae found in each species of snails were fed to experimental hamsters to obtain the adult worm. The adult worms were recovered from hamsters fed metacercariae from F. sumatrensis polygramma and F. doliaris, but not from hamsters fed metacercariae from 3 remaining species. The worm recovery was detailed in Table 3. The 2-week old adult worms were classified into 2 species, including E. revolutum (metacercariae from F. sumatrensis polygramma) and Echinostoma macrorchis (metacercariae from F. doliaris). Their morphology was described as below:
Echinostoma revolutum (see Fig. 2)
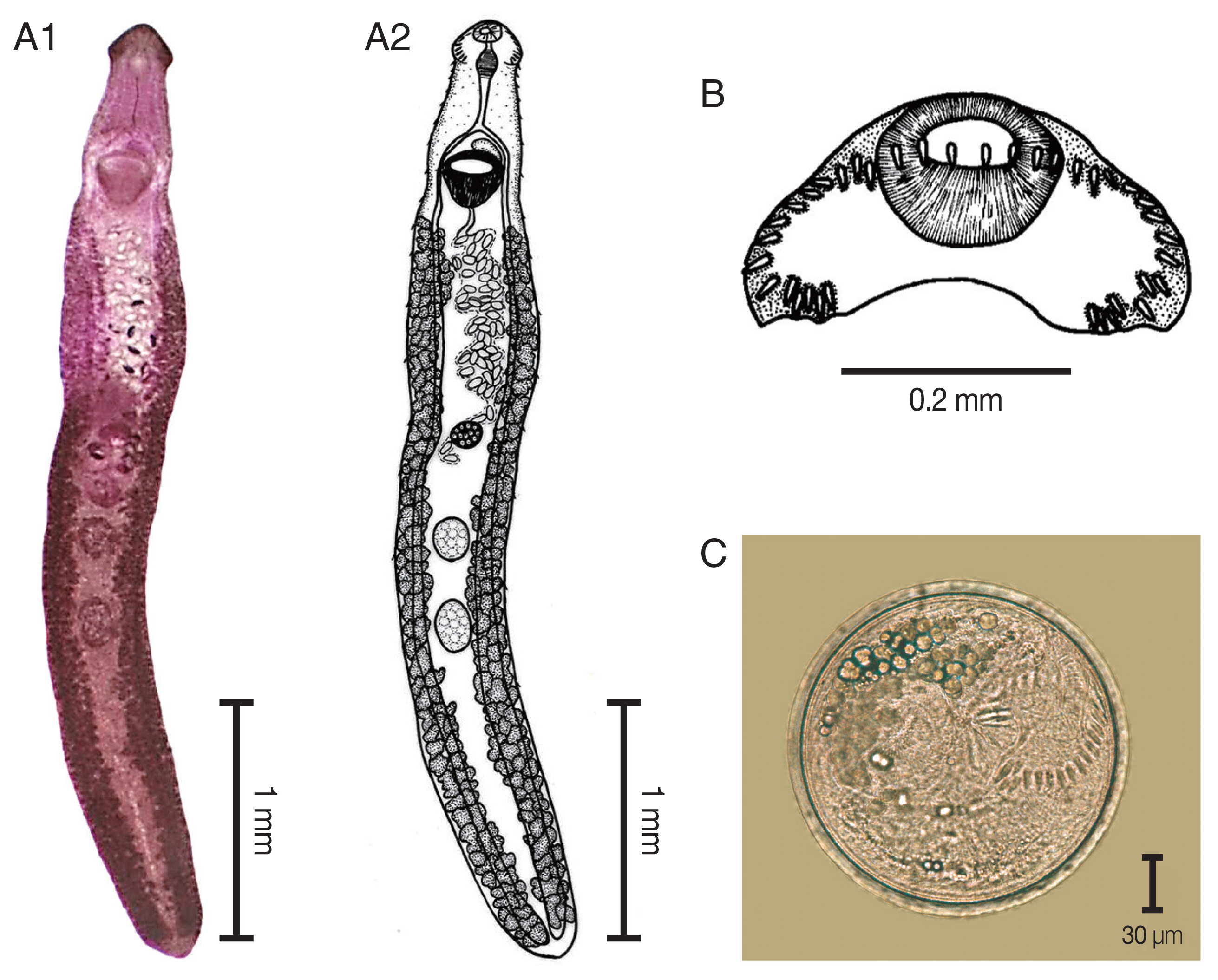
Echinostoma revolutum. (A) The adult stage; permanent slide (A1) and drawing (A2). (B) Head collar revealing 37 collar spines. (C) A metacercaria, spherical in shape with double layer cyst wall and 37 collar spines.
The elongated body of the specimens ranged between 3.8 mm and 8.5 mm in length (5.8 mm average) and exhibited dorso-ventral flattening. The tegument of the body was covered with small spines from the anterior to the posterior of the body. There was an average of 37 collar spines in a semicircle: 5 spines on each side, 6 lateral spines in a single row on each side, and a double row of 15 dorsal spines. The oral sucker was located subterminal and was subspherical in shape. The prepharynx was short. The pharynx was a well-developed muscle. The esophagus was long. The intestinal bifurcation extended from the anterior level of the ventral sucker to near the posterior extremity of the body. The ventral sucker was well developed, muscular, spherical, and larger than the oral sucker. It was located at anterior portion of the body. Two testes were tandem, oval to subspherical in shape, and located in hind body. The cirrus sac was located dorsally between the levels of intestinal bifurcation and anterior level of the ventral sucker. A genital pore was located at median. The ovary was spherical and located in the middle of the body. The Mehlis’gland was located between the ovary and the anterior testis. The uterus was formed by numerous transverse loops between the ventral sucker and the ovary. The vitellarium follicular was formed by lateral fields that were posterior to the ventral sucker and extended to the posterior end of the body. Eggs were operculated, elliptical, yellowish, and thin-shelled. The excretory pore was located at the posterior end of the body.
Echinostoma macrorchis (see Fig. 3)
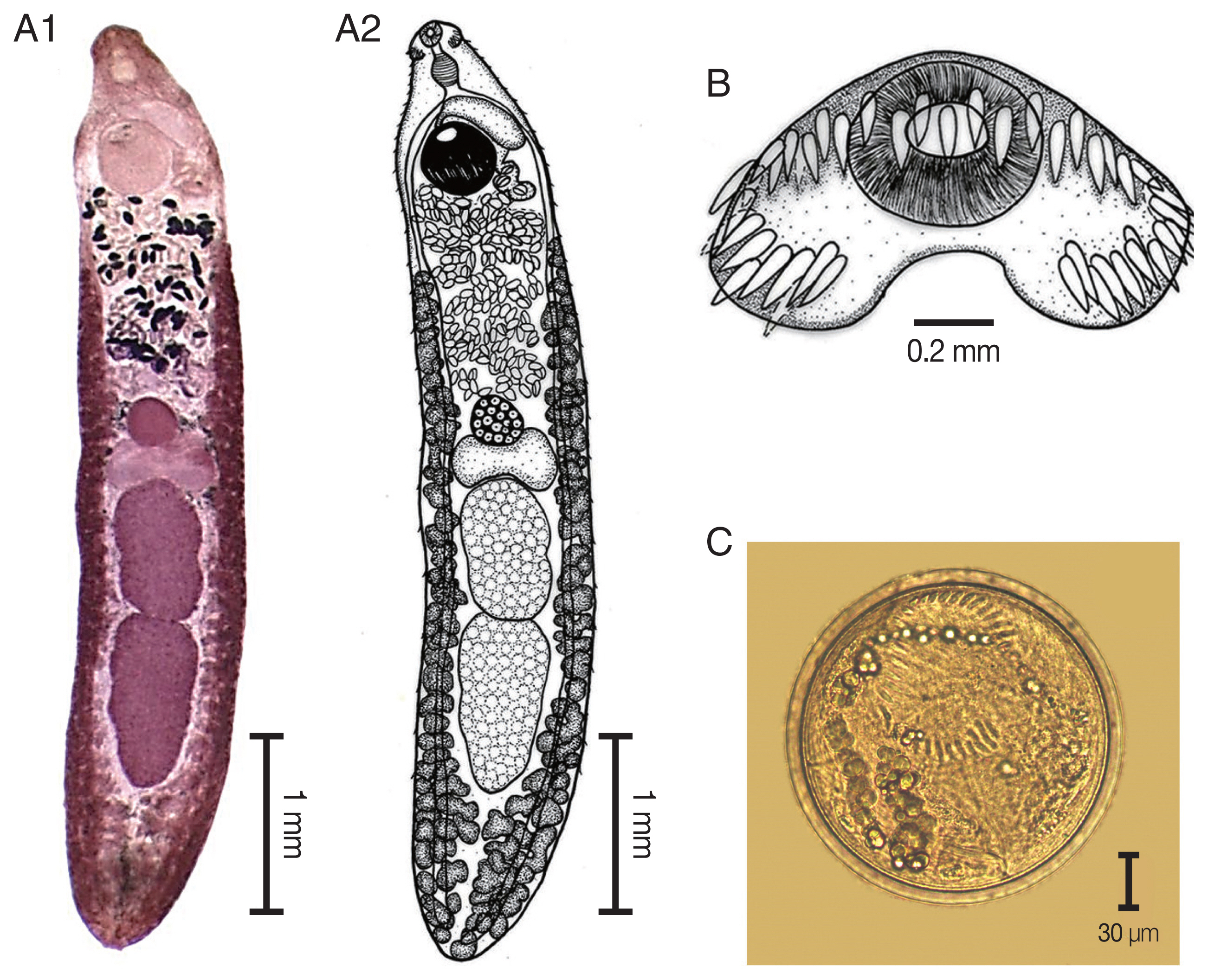
Echinostoma macrorchis. (A) The adult stage; permanent slide (A1) and drawing (A2). (B) Head collar with 45 collar spines. (C) A metacercaria, spherical in shape with double layer cyst wall and 45 collar spines.
The elongated body of the specimens ranged from 4.2 mm to 5.9 mm in length (4.9 mm average) and exhibited dorso-ventral flattening. The tegument of the body was covered with small spines on the anterior of the body extended to the posterior portion of the body. There was an average of 45 collar spines that created a semicircle around collar; 5 spines on each side and 37 spines in double rows (18 oral and 19 aboral). The oral sucker was located at the subterminal and was subspherical in shape. The prepharynx was short. The pharynx was well developed and muscular. The esophagus was long. The intestinal bifurcation was anterior to the ventral sucker and on the posterior end of the body. The ventral sucker was well developed, muscular, spherical in shape, larger than the oral sucker, and located at posterior part of the anterior quarter of the body. Two testes were tandem, large, elliptical, with slight indentations and pointed posterior end of posterior testis, and located in the posterior half of the body. The cirrus sac was a pyriform shape and located dorsally between the levels of intestinal bifurcation and anterior level of the ventral sucker. The genital pore was located anterior to the ventral sucker and opened into the genital atrium. The ovary was spherical and located at midbody. The Mehlis’ gland complex was located between the ovary and anterior testes. The uterus contained shelled eggs and formed numerous transverse loops between the ventral sucker and ovary. The vitellarium follicular formed lateral fields posterior to the ventral sucker that extended to the posterior end of the body. Eggs were operculated, elliptical, and thin-shelled. The excretory pore was located at the posterior end of the body.
Phylogenetic trees based on ITS2 and nad1 sequences
The specimens including metacercarial stages originated from F. martensi martensi (NT2), A. helena (NT3), B. pulchella (NT4), and B. siamensis (ND5), and adult stages obtained from experimental hamsters fed with metacercariae from F. sumatrensis polygramma (NT1) and F. doliaris (NT6) were subjected to DNA sequencing of ITS2 and nad1 regions. The ITS2 products revealed the size of 578 bp and 416 bp in specimens NT1–NT5 and specimens NT6, respectively, whereas the nad1 products revealed the size of partial sequence of 486 bp in all specimens. The ITS2 (388 bp in length) and nad1 (484 bp in length) sequence alignments of our specimens compared with relative species in GenBank, were shown in Fig. 4A and 4B, respectively. The variation of nad1 region was higher than those of ITS2 region.
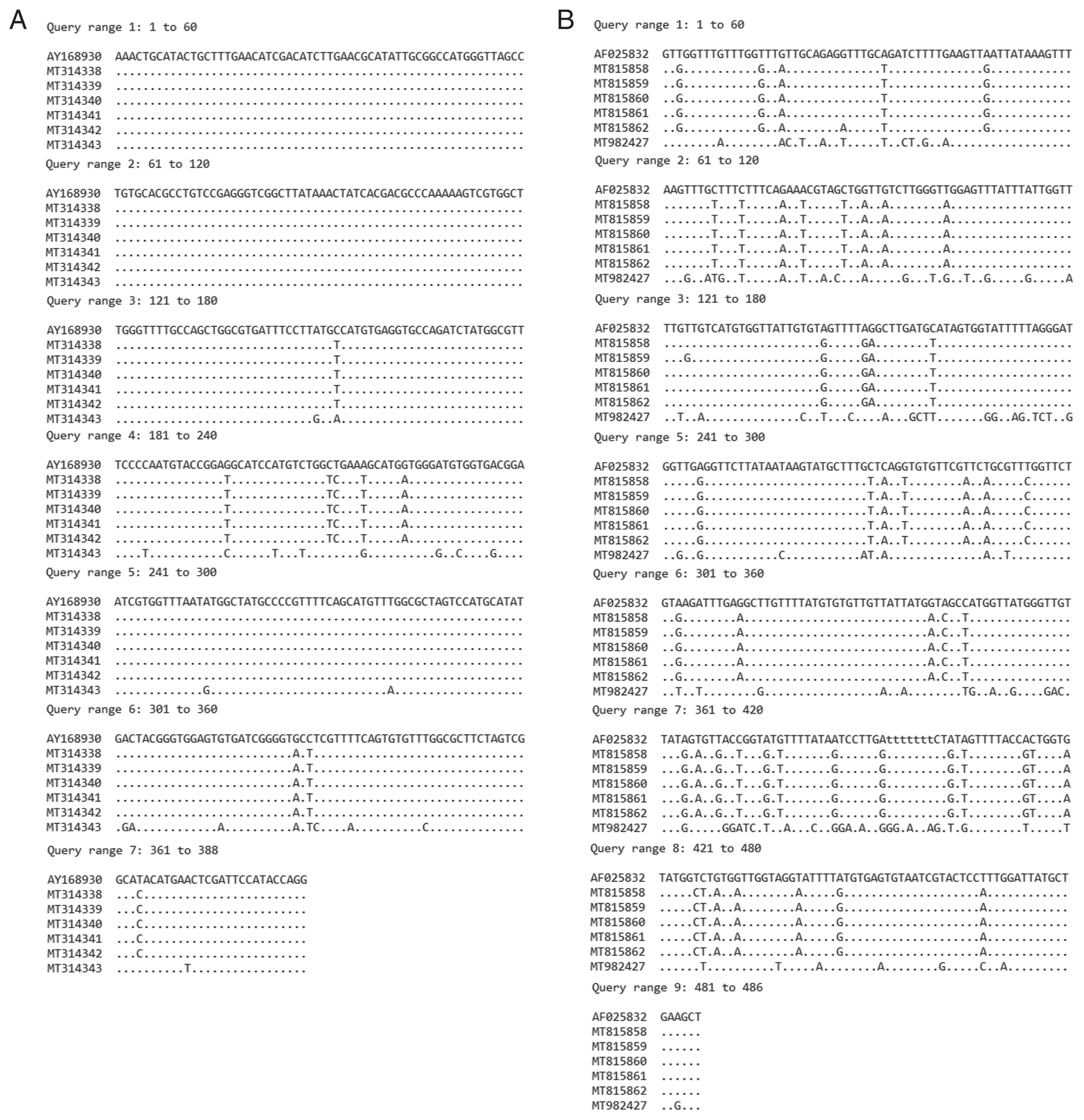
Comparison of sequence alignment of recovered E. revolutum and E. macrorchis with relative E. revolutum sequences in GenBank. (A) ITS2 region. (B) nad1 region.
Both ITS2 and nad1 trees were constructed using a character method (ML) and a distance method (NJ) with bootstrap values of 1,000 replicates. ITS2 tree (Fig. 5A) revealed 4 groups of the Echinostomatidae, including the revolutum group (clade A), Echinoparyphium recurvatum group (clade B), Hypoderaem conoideum group (clade C), and Euparyphium capitaneum group (clade D). E. revolutum found in this study (NT1–NT5) accession no. MT314338–MT314342 were clustered with the ‘revolutum’ group. E. macrorchis from this study (NT6) accession no. MT314343 was grouped with E. recurvatum group.
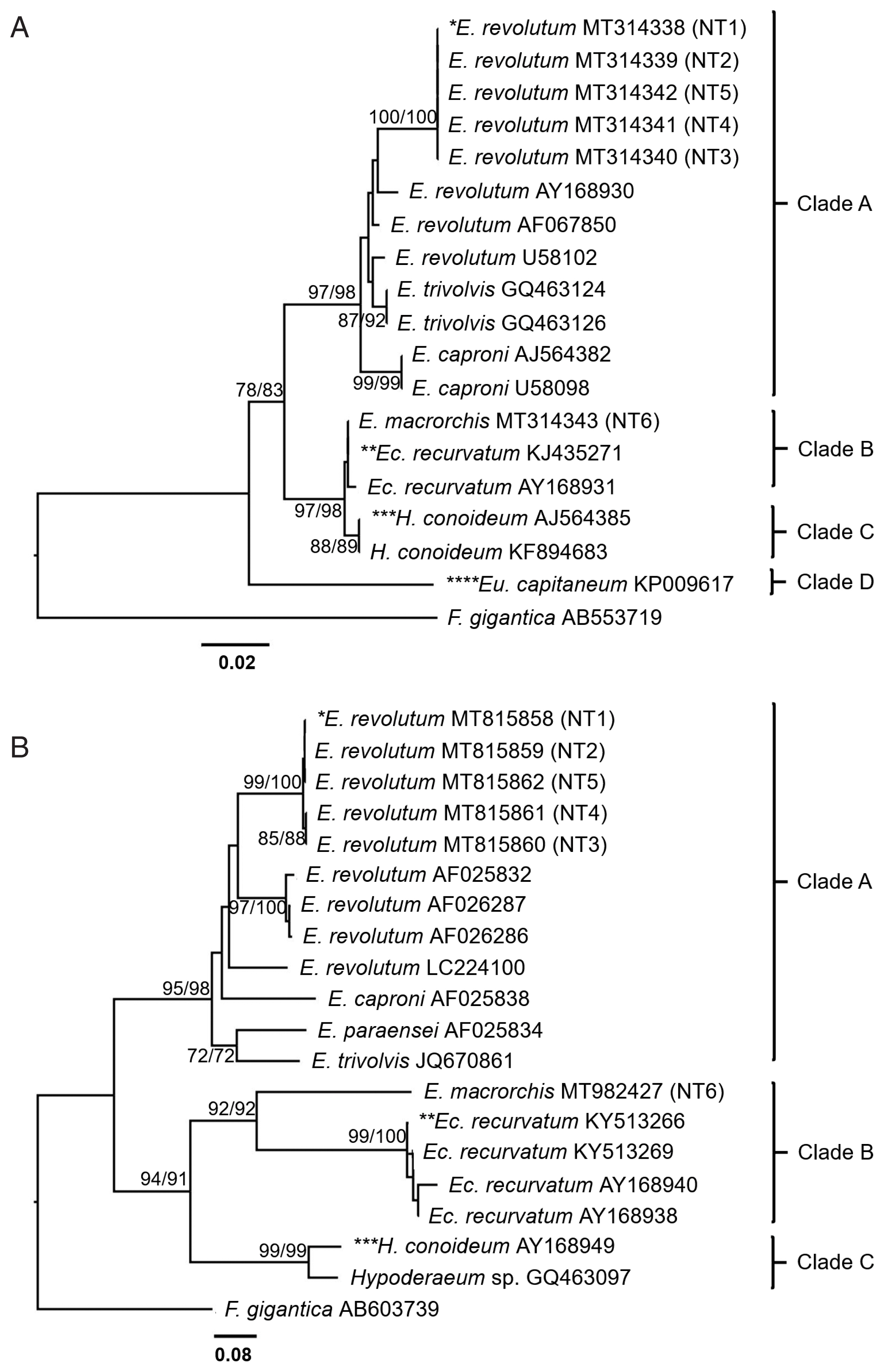
Phylogenetic trees of echinostomes in Upper Northern Thailand constructed based on ITS2 and nad1 sequences using maximum-likelihood (ML) and neighbor-joining (NJ) methods with 1,000 bootstrap replicates (NJ/ML). (A) ITS2 tree. (B) nad1 tree. NT1=E. revolutum adult from an experimental hamster fed the metacercariae from F. sumatrensis polygramma, NT2=E. revolutum metacercaria from F. martensi martensi, NT3=E. revolutum metacercaria from A. helena, NT4=E. revolutum metacercaria from B. puchella, NT5=E. revolutum metacercaria from B. siamensis, NT6=E. macrorchis adult from an experimental hamster fed with metacercariae from F. doliaris.
The nad1 tree (Fig. 5B) revealed 3 groups of the Echinostomatidae, including the revolutum group (clade A), E. recurvatum group (clade B), and Hypoderaeum spp. group (clade C). E. revolutum found in this study (NT1–NT5) accession no. MT815858–MT815862 for nad1 were clustered with the revolutum group. E. macrorchis from this study (NT6) accession no. MT982427 for nad1 was clustered as a sister group to E. recurvatum group.
DISCUSSION
The present study found the infection of echinostome metacercariae in 24 districts from 7 provinces in Upper Northern Thailand with the total prevalence of 40.4%. Only 2 districts, including Pua in Nan and Mae Chai in Phayao Provinces were not positive for echinostome infections. The echinostome infections in snails collected from Nan, Phare, and Phayao Province were first reported. The highest infection was found in Rong Kwang (Phare) followed by Mueang Phayao (Phayao) and Chiang Dow district (Chiang Mai) with the prevalence of 100%, 97.1%, and 78%, respectively. The lowest prevalence was found in Phu Kamyao (Phayao) followed by Mueang Lampang (Lampang) and Mueang Chiang Mai (Chiang Mai) with the prevalence of 5.8%, 10.4%, and 11.4%, respectively.
This study gives the epidemiological information of echinostomes in freshwater snails in 2017–2019 period. There are many possible reasons for different findings in the prevalence and intensity, such as due to the abundance and biodiversity of freshwater snails, levels of water, and the periods during which the specimens were collected. They are factors that facilitate host survival and transmit to the host. According to Noikong and Wongsawad [18], the levels of water during a rainy season are critical for the cercariae to be able to find a secondary host snail. However, there are different prevalence and intensity in each snail. This study agreed to previous studies that reported 7 freshwater snails from Chiang Mai, Chiang Rai, and Lamphun Province in Northern Thailand acting as the second intermediate host of echinostomes, including A. helena, E. eyriesi, Bithynia funiculata, B. siamensis siamensis, F. doliaris, F. sumatrensis polygramma, and F. martensi martensi [17,18,22]. High prevalence and intensity in Filopaludina snails indicated more susceptible to echinostomes than other snails while A. helena was the lowest. B. pulchella was the first record in Upper Northern Thailand as the second intermediate host of echinostomes. Their prevalence was rather high even though the intensity was low. Comparison in the family Bithyniidae, echinostome metacercariae were more prevalent in B. pulchella than B. siamensis siamensis and B. funiculata which were recorded in a previous report [22]. It is interesting to future study the role of B. pulchella as a second intermediate host of echinostomes. In addition, the environmental condition and diversity of freshwater snails as both the first and second intermediate hosts must be further studied particularly in high infection areas.
The echinostome metacercariae originated from this study were morphologically divided into 2 groups based on the number of collar spines. The first group possessed 37 spines and found in F. sumatrensis polygramma, F. martensi martensi, A. helena, B. pulchella, and B. siamensis siamensis. The second group possessed 45 collar spines and found only in F. doliaris. This result was similar to Chantima et al. [17] who found the echinostome metacercariae with 37 collar spines in Filopaludina spp., Bithynia spp., and A. helena from Chiang Mai Province, and identified as E. revolutum based on adult worms. The 2-week-old adult echinostomes were obtained from experimental hamsters fed the metacercariae from F. sumatrensis and F. doliaris, whereas the echinostome metacercariae from 4 remaining snail species did not develop into adults. These different results may have been due to different numbers and degree of maturation of metacercariae force fed to hamsters [32].
In our study, the echinostome adults were classified into 2 species, E. revolutum and E. macrorchis. The number of collar spines is a unique characteristic of echinostome flukes that can be used for species identification [5,33,34]. The adult E. revolutum obtained in this study revealed 37 collar spines, while E. macrorchis presented 45 spines that is consistent with the number of collar spines in metacercarial stage. There are various 37-collar-spined Echinostoma spp. belonging to the ‘revolutum’ group, including E. caproni, E. echinatum, E. friedi, E. jurini, E. luisreyi, E. miyagawai, E. paraensei, E. parvocirrus, and E. trivolvis [35,36] but they are different in the morphology, host-parasite relationships, and geographical distribution [37–39]. E. revolutum differed from other species in its cirrus sac extending to middle of the ventral sucker and smooth testes. However, E. revolutum closely resembled E. jurini, although they were different in their definitive host. E. jurini was found only in mammals but E. revolutum was found in both mammals and birds [38,40,41]. E. revolutum has been shown to endemically infect humans in Southeast Asia [7,42]. In Thailand, it has been found in Northern, Northeastern, and Central parts, i.e., the metacercarial stage from freshwater snails and adult stage from poultry [9,10,17,20,21].
E. macrorchis was first found naturally in Rattus norvegicus and R. rattus in Japan [43]. Then, this echinostome has been recorded in other rodents, birds, and humans in Japan [44–47]. The presence of this echinostome was also reported in Taiwan, Korea, and Lao PDR [48–51]. E. macrorchis recovered in this study was a new record in Thailand. There are 11 Echinostoma species bearing 43–45 spines: E. academica, E. aegyptica, E. attenuatum, E. australasianum, E. azerbaidjanicum, E. coromandum, E. coronale, E. dietzi, E. gotoi, E. macrorchis, and E. phasianina [52]. The body length of our specimens did not exceed 6.5 mm, like in E. academica, E. aegyptica, E. attenuatum, E. macrorchis, and E. phasianina, while E. australasianum, E. azerbaidjanicum, E. coromandum, E. coronale, E. dietzi, and E. gotoi exceed 13.0 mm. However, our specimens differed from E. academica that has a larger head collar. The testes morphologies in our specimens, i.e., large, elliptical, with slight indentations and a pointed posterior end of posterior testis, were highly consistent with those of E. macrorchis [43,53]. Whereas, E. aegyptica has smooth surfaced and rounded posterior end of the posterior testis [54]. The testes of E. attenuatum and E. phasianina are small, slender, and elongated. The morphologies and measurements of our specimens agreed well with previous descriptions of E. macrorchis (Table 4). This study first confirmed that F. doliaris collected from Upper Northern Thailand were infected with E. macrorchis metacercariae. E. macrorchis metacercariae have been previously found in Cipangopaludina japonica from Japan [55], in Cipangopaludina sp. from Vientiane Municipality, Lao PDR [49], and in Cipangopaludina chinensis malleata snails from Korea [50] and Xiengkhuang Province, Lao PDR [51]. Moreover, various other snails, i.e., Assiminea, Biomphalaria, Bulinus, Corbicula, Gyraulus, Hippeutis, Lymnaea, Physa, Segmentina, and Thiara, and amphibians, i.e., Rana spp. and Hynobius sp., have been recorded as the second intermediate hosts of E. macrorchis in Japan and Taiwan [53,56]. Two morphological features of E. macrorchis, including 5 corner spines and large quadrangle-shaped testes with irregular surface are different from those of E. recurvatum (possessing 45 collar spines). E. recurvatum has 4 corner spines and small and oval testes with smooth surface [57]. Rats and mice are suitable as experimental definitive hosts of E. macrorchis [53], while ducks and chicks are suitable for E. recurvatum [57].
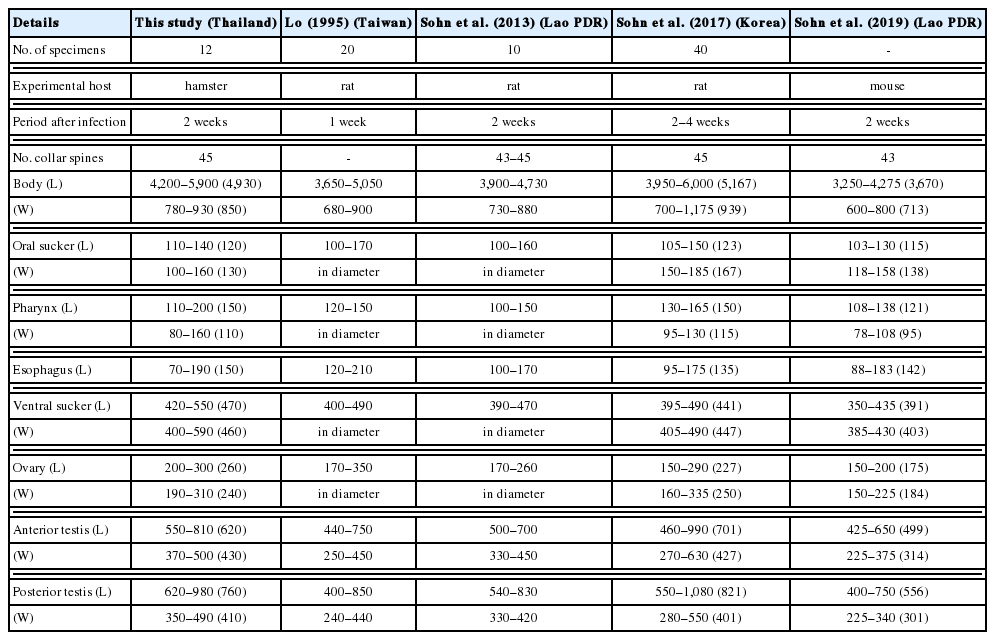
Comparative features and measurements (μm) of Echinostoma macrorchis adults recovered from various experimental hosts infected with the metacercariae
Both ITS2 and nad1 sequences could confirm that our specimens (NT1–NT5) were E. revolutum. The query sequence of E. revolutum in both metacercarial and adult stages were closely related to E. revolutum from the European strain and separated from other species in the ‘revolutum’ group (Fig. 5A, B). The morphological characteristics of the adult stage were consistent with the molecular characteristics. Although the 37-collar-spined echinostome metacercariae recovered in each species of snails were identified as E. revolutum based on ITS2 and nad1 sequences in this study, the morphology of adult flukes should be further studied for species reconfirmation. This study indicated that the snails collected from Upper Northern Thailand were infected with 1 echinostome species/snail species. However, large numbers of metacercariae should be used for future studies because individual snails may be infected with more than 1 echinostome species.
Both ITS2 and nad1 sequences of E. macrorchis (45-collar-spined) were separated from those of the ‘revolutum group’ (37-collar-spined). The number of collar spines is the main morphological characteristic associated with the phylogenetic relationships [28,58]. Besides, the characters of the testes, position of the cirrus sac, distribution of the vitellaria, host-parasite relationships, and geographical distribution indicate the phylogenetic relationships of echinostomes [27,30,31]. In our study, E. macrorchis was clustered with 45-collar-spined group; however, E. macrorchis differs from E. recurvatum in the number of corner spines and suitability of experimental definitive hosts. The nad1 sequence could support that E. macrorchis was separated from E. recurvatum with high bootstrap support (Fig. 5B). However, there had been no genetic information of E. macrorchis in previous studies, and we confirmed that the 45-collar-spined metacercariae were E. macrorchis based on the adult morphology. The main characteristics of 5 corner spines, quadrangle shaped testes, irregular testis surface, and pointed posterior end of the posterior testis differed from other echinostomes bearing the same 45 collar spines. However, the phylogenetic relationships of E. macrorchis with other 45-collar-spined echinostomes must be further studied.
ACKNOWLEDGMENTS
We would like to thank Dr. Nattawadee Nantarat and Ms. Atcharaphan Wanlop for use of their facilities, and the Research Center in Bioresources for Agriculture, Industry and Medicine, Faculty of Science, Chiang Mai University for financial support. This research was partially supported by Chiang Mai University, Chiang Mai, Thailand. Special thanks are extended to www.proof-reading-service.com for English correction.
Notes
CONFLICT OF INTEREST
We have no conflict of interest related to this study.